Research Themes
Our activities mainly focus on interdisciplinary approaches to solve various problems of health and diseases that come under mechanobiology. To solve these problems, we are engaged on but not limited to the following fronts:
Mechanical Roles of Chaperone-assisted Protein Folding Events
Protein folding under force is an integral source of generating mechanical energy in various cellular processes, ranging from protein translation to degradation. Chaperones are specialized proteins known to interact with proteins under mechanical force and regulate their folding. But, how they respond to force and control cellular energetics remains elusive. To address this question, we introduce microfluidic magnetic-tweezers technology to mimic physiological force environment on substrate proteins, keeping the chaperones out of force-influence. Previously we have reported the redox-switchability of E.coli DsbA in the presence of mechanical force. We found chaperones behave differently, while these client proteins are under force than its previously known functions. We have managed to classify the chaperones as foldase (those who favors the folding) and holdase (those who favors the unfolding) and neutral (favors neither), according to their mechanical behavior under force. Further, we are expanding our study to various eukaryotic chaperones that might work under force as well as the bacterial periplasmic chaperones to decipher their mechanism in force-influenced protein-folding
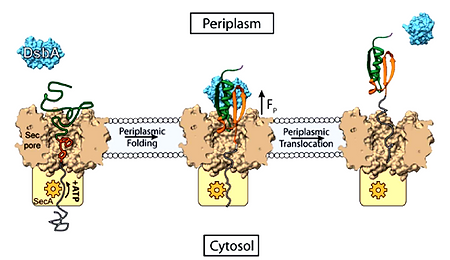
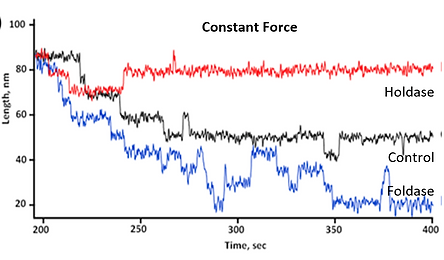
Role of Mechanosensitive Proteins in Focal Adhesion-mediated Cellular Processes
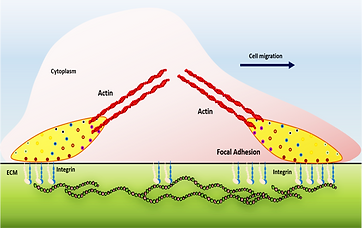

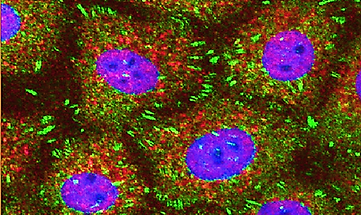
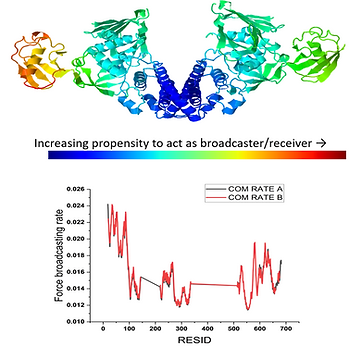
Cell migration is controlled by a force-driven cellular brake system called focal adhesion (FA), a multiprotein assembly of almost 200 proteins. As a well-defined clutch-accelerator system, the proper functioning of FA depends on its constituent mechanical linkages, which both transmit and transduce the mechanical force into the cells. These FA proteins interact mechanically and control the cell migration through traction force. Furthermore, these mutual interactions modulate the force-sensitivity of these proteins, which becomes dysregulated in different pathological conditions. Therefore, it is of utmost importance that what plausible factor can modulate this force-response of these proteins? Since each single protein molecule has its own contribution to the overall FA dynamics and thereby the cell migration, the force response of a single protein is of prime importance to understanding the underlying mechanism. Single-molecule techniques are very handy in knowing their mechanical response and that becomes our strength. Therefore, we study these proteins’ mechanical stability, how their post-translational modifications affect it, and how protein-protein interactome builds a mechano-regulatory network.
Mechanosensitive proteins play a crucial role in focal adhesion-mediated neurodegeneration, a process that involves the breakdown of nerve cells in the central nervous system due to abnormal mechanical forces acting on the cells. Focal adhesions are multi-protein complexes that connect the cell's cytoskeleton to the extracellular matrix, allowing the cell to sense and respond to mechanical cues from its environment. In the context of neurodegeneration, these mechanosensitive proteins become instrumental in transmitting and translating mechanical signals, ultimately contributing to neuronal damage. Understanding the role of mechanosensitive proteins in focal adhesion-mediated neurodegeneration is critical for developing targeted therapeutic interventions. By modulating the activity of these proteins or their downstream signaling pathways, we aim to mitigate the cellular responses to abnormal mechanical forces, potentially slowing the progression of neurodegenerative diseases and offering new avenues for treatment and prevention. Furthermore, this knowledge highlights the importance of considering mechanical factors alongside biochemical and genetic aspects in the study of neurodegeneration, broadening our understanding of the complex mechanisms underlying these devastating conditions.
​Developmental Mechanobiology and its Intersection with Cancer
From the beating heart to the growth of our brain, mechanical forces play a pivotal role in sculpting life itself. Developmental mechanobiology is a field of study that explores the mechanical forces and physical interactions governing tissue development and homeostasis during an organism's growth and regeneration. It investigates how mechanical cues, such as tension, compression, and shear forces, play pivotal roles in shaping and directing cellular processes, including proliferation, differentiation, and tissue organization. We employ a range of techniques, such as covalent magnetic tweezers to study mechanochemical signaling, microfabricated substrates to apply controlled mechanical forces, and live-cell imaging to observe cellular responses in real time. With this interdisciplinary approach, we aim to decipher the intricate relationship between mechanical signals and the underlying molecular and cellular processes in development.
Understanding the stochastic nature of the mechanome is crucial for appreciating the variability in biological responses to mechanical cues, which can have implications for various physiological processes, including tissue development, wound healing, and disease progression. We use mathematical models, computational simulations, and artificial intelligence to study and predict the stochastic behavior of mechanosensitive processes, helping to unravel the complexity of mechanobiology.
One of the significant intersections between developmental mechanobiology and cancer lies in studying the mechanical regulation of cancer stem cells. Cancer stem cells are a small subset of cells within a tumor that possess stem cell-like properties, including self-renewal and differentiation capabilities. These cells are responsible for tumor initiation, progression, and resistance to therapy. We try to investigate how mechanical cues within the tumor microenvironment influence the behavior of cancer stem cells. Understanding the impact of mechanical forces on these cells is crucial, as it can provide insights into their maintenance and propagation. Techniques like microfluidic devices and 3D culture systems enable us to recreate the complex mechanical conditions within tumors and study how they affect cancer stem cell behavior. These insights are vital for developing targeted therapies that disrupt the mechanical cues sustaining cancer stem cells and, in turn, hinder tumor progression and improve treatment outcomes.

Role of Small Molecule Drugs and the Micro-environment in Regulating Protein Mechanical Properties
Exploring the dynamic interplay between small-molecule drugs and the cellular microenvironment in regulating the mechanical properties of proteins is a compelling area of mechanobiology research. This investigation delves into how pharmaceutical compounds, known as small-molecule drugs, can impact the mechanical characteristics of proteins in the context of the microenvironment. Protein mechanical properties are vital for their role in cellular structure and function. Small-molecule drugs, designed to target specific cellular processes, can alter protein mechanics by binding to or modifying them, influencing their conformation, stability, and interactions. Furthermore, the microenvironment, encompassing factors like the extracellular matrix, cell interactions, and mechanical cues, significantly shapes how proteins respond to small-molecule drugs and mechanical forces. This research is invaluable for potential applications in drug development and tissue engineering, as it unravels the complex interactions between small-molecule drugs, the microenvironment, and protein mechanics, offering insights into therapeutic design and the manipulation of protein mechanics for clinical and biological advancements. We utilize a range of cutting-edge methods to investigate how existing medications influence the mechanical integrity of diverse focal adhesion proteins, intending to repurpose these drugs. Additionally, our state-of-the-art techniques empower us to meticulously examine the unintended consequences of these medications on structural proteins.
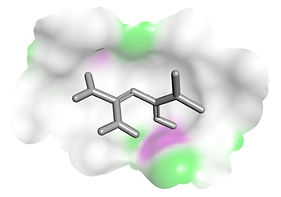
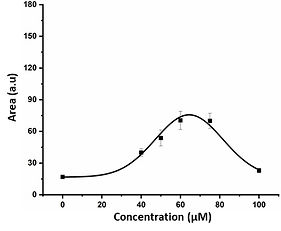
